A better understanding of how brain systems interact could help in the fight against Alzheimer’s disease
How do neuromodulatory subcortical systems interact in the course of learning? This is the question Balázs Hangya, the leader of the Lendület Laboratory of Systems Neuroscience in the Institute of Experimental Medicine of the Hungarian Academy of Sciences (MTA-KOKI) is attempting to answer. He has just been awarded a 1.5 million euro grant for his research project by the Starting Grant Program of the European Research Council (ERC). In the long run, his research can help in the understanding of the cognitive disorders characterising Alzheimer’s and Parkinson’s disease.
10th October, 2016
“One of the most crucial prerequisites in winning such a grant is that the scholar presents a really important, relevant and exciting research question which addresses a topical problem and can be answered with our present arsenal of knowledge and devices,” says Balázs Hangya, whose research results concerning neurodegenerative diseases caused by functional cognitive disorders such as Alzheimer’s or Parkinson’s disease are very promising. “The treatment of these patients is a burden for both the healthcare system and the families affected. The number of patients with Alzheimer’s is estimated at around 35-40 million globally, whose treatment costs an average of 600 billion dollars per year,” the researcher claimed.
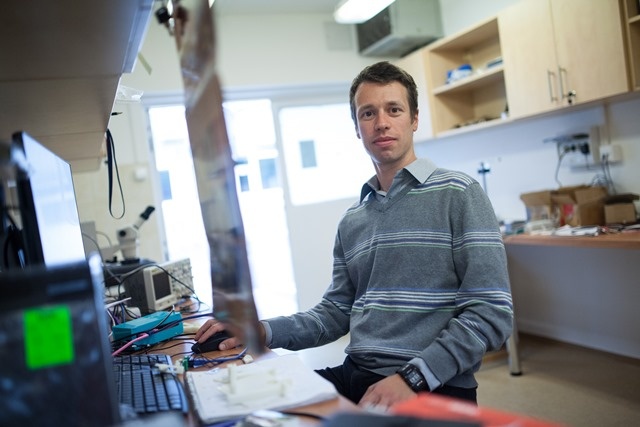
Balázs Hangya is convinced his success is partly due to the Academy’s Momentum (Lendület) Program, which enabled him to found his own research group in the Institute of Experimental Medicine of the Hungarian Academy of Sciences (MTA-KOKI) over the course of 2015.
“A grant like this from the Momentum (Lendület) Program proves that the scholar is able to acquire independent resources – even in the phase of his research career in which he has just established his first laboratory and has not proven his abilities as a leading researcher to the wider public,” added Mr Hangya.
Discovery of “controlling mechanisms” below the cortex
This research focuses on the learning process, which explains why diseases severely affecting cognitive processes are also studied here. “Learning can be defined as the process by which we remember new pieces of information. Memory, in turn, is the storage and recall of data. Areas beneath the cortex take part in both learning and memory processes. The most important roles are played by regions functioning through different neuromodulatory compounds: the communication in these regions is governed by molecules or modulators which do not belong to either inhibitors or excitors. The way these function is more complicated and complex and occur on several time scales simultaneously,” the scholar explained.
The best known neuromodulatory systems are the following: dopaminergic; cholinergic; serotonergic; and noradrenergic. Besides these, several other neuromodulatory systems are present in the brain, which have not yet been explored to a wide degree by neurobiologists. To start, Mr Hangya and his colleagues focus on two of the classical neuromodulatory systems: on the cholinergic system that plays a crucial role in learning; and the dopaminergic system, which is an important part of the reward system, and as such takes part in the process of strengthening knowledge. Researchers study how these systems interact in the learning process. In the long run however, Mr Hangya aspires to enlarge the scope of the study to other neuromodulatory systems, primarily to the serotonergic and noradrenergic systems.
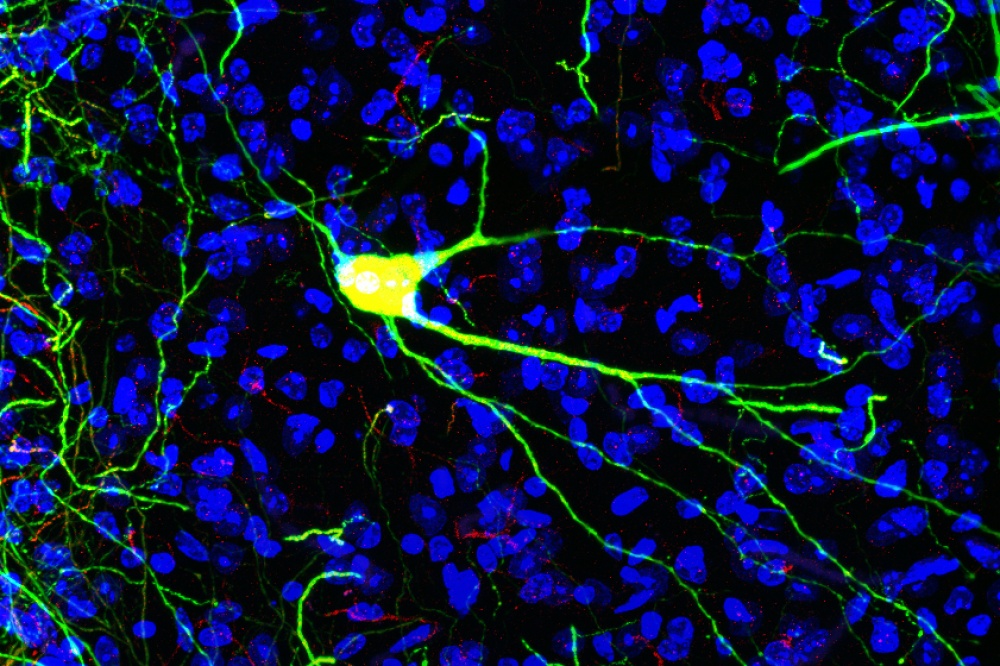
“Why so many systems governing similar cognitive functions exist is an exciting question. Neuromodulatory systems – in our case, the cholinergic and the dopaminergic systems – both carry information on reward, punishment and prognosis. These are all useful factors concerning learning. Although it is a crucial question, we still do not know how these similar pieces of information lead to different processes in the cholinergic and dopaminergic systems. To achieve this, our novel approach examines the two systems separately. I know of no earlier study in which the two neuromodulatory systems were studied in the same “learner” mouse,” said the researcher.
Reward or punishment? How do mice learn?
“We use different transgenic, that is, genetically-modified, mouse strains for our optogenetic experiments. The genome is modified in such a way that specific proteins are expressed in the dopaminergic and cholinergic cells, enabling us to identify these cells over the course of the experiment. The activity of the cells is transported and measured while the mouse is busy with the tasks,” explained Mr Hangya.
He recounted that mice are taught different tasks, all including the learning of something new. For example, some sounds signal reward, while other sounds signal punishment. Sound stimuli completely new to the mice are given from time to time. The mouse has to learn whether the new sound promises reward or punishment.
To catch the “mathematician in the brain” red-handed
“Our brain is designed to provide prognoses. It is an automaton in the mathematical sense, whose task is to predict future events with the highest possible probability. In the case of learning, this is especially true. Dopaminergic cells react very well to predictive stimuli, that is, stimuli that predict a reward or its absence. In many cases, however, when the reward correctly predicted by the stimulus finally appears, dopaminergic cells display no reaction as the brain already knows the availability of the reward beforehand. Therefore, a considerable part of the experiment was transformed into a mathematical model,” explained the scientist. “A pronounced aim of our project is to catch the mathematician living in our brain red-handed. We hypothesise that it is responsible for a much wider range of tasks than the pure prognosis of decision confidence. Our aim is to examine the role of this mathematician in the learning process and to find out how to describe the behaviour of neuromodulatory systems by mathematical models. I hope we can discover fundamentally new facts by the end of our five-year-long project: the cooperation and governing mechanisms of the dopaminergic and cholinergic systems during the learning process. We are already aware of the fact that these two systems operate on different time scales. It is also clear that they often carry very similar pieces of information. It is still to be investigated as to why information appears on different time scales in the case of the two different neuromodulatory systems. Another question is whether it is the same piece of information that appears and is spread on towards different parts of the brain, or whether these are in fact similar reactions to different pieces of information.”
What do we need to know about optogenetics?
Optogenetics, which is based on photosensitive proteins, has revolutionised neuroscience. Genetically-modified neurons are made to fire by short laser impulses. This method can switch on or switch off any designated part of the nervous system. Based on such observations, the exact role of certain parts of the brain and of certain cell types in different neurophysiologic processes can be identified.